Towards a Microscopic Model for Species Transport in Lithium-Sulphur Cells
Lithium-sulphur (Li-S) batteries have the potential to surpass the energy storage capability of Li-ion batteries due to their high theoretical gravimetric energy density of 2700 Wh/kg. However, the processes which drive the system behaviour are much more complex than those in a Li-ion cell, meaning that controlling them to realise energy densities much above 350 Wh/kg is challenging.
In a Li-S cell, solid phase sulphur, S8, is electrochemically reduced during cell discharge to sulphur in the form of lithium sulphide precipitate, but for this to occur there are a series of electrochemical redox steps, chemical reactions and precipitation and dissolution processes, all linked and offering possible feedback loops [1]. Unfortunately, the inability to experimentally probe many of the processes individually in situ means that neither the processes nor how they interact are fully understood. By using both experiments and mechanistic models we can try to determine what microscopic processes dominate under certain conditions and how these combine to control the macroscopic cell behaviour during operation, for example the shape of the discharge voltage curve.
Mechanistic models for Li-S cells have previously been developed in which the cell structure is homogenised, electroneutrality is assumed and the cell is treated in a one-dimensional manner, similar to existing approaches used for Li-ion cells [2, 3]. However, the aforementioned complexity means that such homogenised models may not be entirely appropriate for describing certain aspects of Li-S cell modelling. Species interactions and the electrolyte structure (i.e. ion concentration profiles) in the pores strongly determine ion behaviour and the rates of reaction, but these properties are lost in the process of homogenisation. By retaining this detail in a high fidelity model we aim to be able to better inform the approximations made in homogenised and lower fidelity models.
We are developing modified Poisson-Nernst-Planck models in one and two dimensions in which ion volume and redox and chemical reactions are accounted for and in which the electric double layer is resolved. We are using COMSOL Multiphysics® software to solve the resulting equations, as shown for example in Figure 1, where the predicted equilibrium concentration profile across a short pore structure is plotted, and in which co-ions, neutral species and the larger counter-ions can be seen to be excluded from the electrode surface located at y=0.5μm. This leads to a model system in which the cell potential naturally arises from the redox reactions moving towards equilibrium and in which the mixed potential from all concurrent redox reactions can be described, shown for example in Figure 2. From this, we can look at how the microscopic properties change as chemical reactions take place or an external current flows between the electrodes, and then from this we can begin to investigate how the microscopic properties of the system can affect its macroscopic (and experimentally measurable) behaviour.
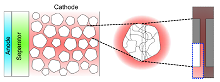
Herunterladen
- minton_presentation.pdf - 1.01MB
- minton_abstract.pdf - 0.22MB