Ionic Neuromodulation and Neurosensing Platform for In Vitro Studies
Overview We successfully simulated a template iontronic system for closed-loop neuromodulation and neurosensing. The model’s goal is to support the careful design of novel platforms for in-vitro micro-electrode-array sensors coupled to conductive polymer-based (CP) ionic actuators used for iontronic neuron stimulation. The remarkably different physical nature of the system’s components, and the strong impact of the microscopic phenomena on the system macroscopic physical properties enforce the adoption of a multiscale and multiphysics approach. The system is formed by a simplified elliptical neuron soma engulfing a mushroom-shaped sensing microelectrode and in proximity of a ring shaped stimulation electrode with polymer coating. Cylindrical coordinates allow us to save remarkable simulation time. The system elements are bathed in a physiological electrolyte containing the relevant mobile physiological ions and fixed organic molecules (described as a fixed charge density).
Interfaces: The interfaces used in the final model are: Electrostatics, Transport of Diluted Species, their multiphysics coupling, and ODEs in keypoint surfaces. The novelties involve four main areas: The workhorse Poisson-Nernst-Planck (PNP) consists of a manual multiphysical coupling between one electrostatic and multiple transport of diluted species (one for each ion species) in the intra- and extracellular electrolytes separately, which entails solving two PNP systems of equations independently. The static and dynamic behavior of the neuron membrane is enforced via ODEs at each membrane’s grid point following the Hodgkin-Huxley formalism [Hodgkin1952] for all ions crossing the membrane [Golomb2006]. We self-consistently update the ionic reversal potentials based on actual ion concentrations at the membrane interfaces. A two-phase model of the CP coating is implemented following [Tybrandt2017]. Each phase in the same geometrical domain is associated with its own electrostatic potential; therefore, the coupling between the physics must be manual. We utilized a space charge density node and a potential coupling to enforce a volumetric capacitance relation between the phases. A dynamic site binding phenomenon is implemented on metal oxide surfaces via ODEs comprising the time constants of the binding reactions.
Results The controlled release of [Ca2+] during an ionic neuromodulation stimulus (see figure, 3D view) effectively stops the bursting activity of the neuron (panel a)), bringing it back to tonic firing (panel b)). The sensed biphasic potential on the electrode appears both without (panel c)) and with the actuation signal, when artifacts superimposed to the useful signal are visible (panel d)).
Conclusions The use of two distinct PNPs allows for direct access of the transmembrane potential avoiding the use of resource-hungry extrusions, which are often unmanageable when the geometry is scaled in complexity. At the same time, the introduction of multiple electrostatic potentials and the manual coupling of the physics enables the investigation of promising materials for ion release/capture (e.g., PEDOT:PSS). Finally, ODEs ease the inclusion of surface phenomena such as membrane ionic fluxes and dynamic site binding at the sensing electrode.
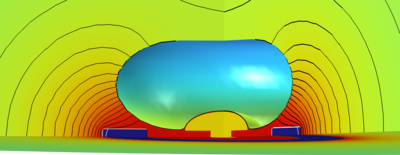
Herunterladen
- Nicolini_5631_poster.pdf - 3.32MB