Modeling On-Chip Nanoscale Trap and Enhance Device for Quantum Photonics Using COMSOL Multiphysics®
In recent years, with the rise of quantum optical technologies, there has been a spurring research interest growing on single-photon emitters (SPE). To date, the focus in engineering improved SPE has been mainly towards better enhancement overlooking the tenuous manufacturing process that demands manual placement of the nano-emitters [1]. To find a scalable approach for realizing SPEs, in this work, we modeled a waveguide base design that will simultaneously trap and enhance emission from nano-emitters using the concept of electro-thermo-plasmonic (ETP) effect [2]. In general, the ETP process involves photo-induced heating of a plasmonic nanoantenna with an applied a.c. bias inside suspension of particles which can initiate rapid micro-fluidic motion and thus enables on-demand particle transport with rapid velocity. To evaluate the multiphysics aspects crucial to the feasibility of the proposed model in COMSOL®, we have used the Wave Optics Module and Heat Transfer (HT) Module to calculate the optical force, Purcell enhancement (PE) factor, and for measuring temperature change associated with electromagnetic heating respectively. Using the concept of plasmonic mode coupling and enhancement of evanescent fields [3], a hybrid plasmonic-photonic mode is excited in the Si waveguide by placing a metal (Titanium Nitride - TiN) over it with a nanoscale gap between them (Figure 1). Absorption of propagating photons by the TiN raises its temperature which enables the ETP process. As apparent from Figure 2, the enhancement of the electric field within the gap region creates a field gradient with the surrounding. Assuming the surrounding is a liquid medium (water) with nano-emitters as particles suspended in it, once brought sufficiently close to the hybrid waveguide by the ETP flow, the field gradient will exert optical force on non-uniformly polarized particles nearby the gap and thus will transport them into the gap region where the electric field is maximum [4]. The force exerted on the particles and associated trapping potential (Figure 3) is analyzed by integrating Maxwell’s surface stress tensor values and this helps to verify the stable trapping capability of the model (≥10KBT) [5]. The trapping strength also depends on perturbing thermal force associated with the temperature-induced density gradient of the liquid medium and this is desired to be not large enough to cause unintended convection. Because of this limitation, the surrounding liquid has been considered as stationary and thereby ‘thermally’ solid in HT in solid studies. Lastly, to get a preliminary measure of PE (the fractional increase in total emission rate) for the model, we calculated the ratio of the radiated power from a point dipole placed within the gap region to free space radiation by measuring the power flux through the cross-sectional area across the wave propagation direction. Given the absence of closed cavity in our model (Q≈1), PE factor is found to be around 6 which seems consistent as per the system. Thus, this Multiphysics study provides a preliminary idea on the feasibility of the project and leaves room for further optimization of the model.
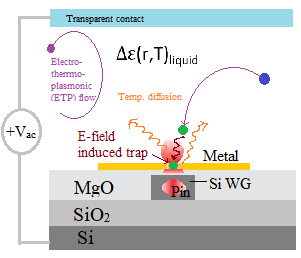