Modeling of Mass Transport in 3D Vascularized Porous Tissue Scaffolds
Porous scaffolds of natural and synthetic polymers and ceramics, fabricated by electrospinning, salt leaching and 3D printing, are widely used for tissue engineering and regeneration (1). The geometric characteristics of a porous scaffold, such as pore size and embedded channels, are important design parameters. Particularly, vasculature-like perfusable channels are critical to convection-based mass transport, which, in sharp contrast to diffusion-based mass transport, significantly improves the transport of oxygen and nutrients to meet the needs of cellular metabolism in thicker tissues with clinically relevant sizes. Computational simulation has been increasingly adopted to help in the design of porous scaffold geometry because it can predict experimental results with reasonable accuracy, thus reducing a large amount of time-consuming and expensive experimental iterations. However, conventional computational simulations are usually based on either a single straight channel or parallel channels; over-simplified geometries do not match the curved and entangled morphologies found in sophisticated natural vasculature or in 3D printed vasculature (e.g., mathematical space-filling Hilbert curve) (2). Here, we employed the CFD Module of COMSOL Multiphysics® simulation software to model a 2D square cross-section of a porous scaffold with embedded perfusable channels in the shape of Hilbert curves. We used Navier-Stokes and Forcheheimer-corrected Brinkman equations to describe liquid flows in perfusable channels and porous matrices, respectively. Cells are seeded only in the porous matrix. The combination of Fick’s law and Michaelis-Menten reactions was used to describe the temporospatial profiles of oxygen and glucose. To account for cellular necrosis, we added a smoothed Heaviside function to terminate the oxygen consumption when oxygen concentration was below a critical level. The porosity and permeability were experimentally obtained using a silk fibroin protein sponge (3). Figure 1 shows the oxygen profile within the scaffold (the initial value is zero), 81 seconds after perfusing culture medium with 0.2 mol/m3 oxygen at 0.002 m/s. The oxygen will first become saturated at the lower-left quadrant. The developed simulation is particularly advantageous to optimize the geometry of scaffolds, including porosity, permeability and vasculature, for culturing a specific cell type(s) and tissues. Furthermore, vasculature system is versatile to provide a consistent or a gradient concentration profile. To better reflect the complexity of 3D printed vasculature and comparing the efficiency of mass transport, the Hilbert curve can be readily replaced by other mathematical curves like bicontinuous cubic lattice, as well as by digitally reconstructed natural vasculature like the capillary network around a pulmonary alveolus.
Figure 1: Profile of oxygen concentration (mol/m3) within a porous scaffold with Hilbert curve-alike vasculature after perfusion of culture media for 81 seconds. White lines indicate velocity streamlines. The square cross-section and the channel is 14 mm and 0.5 mm wide, respectively.
References
1. V. Karageorgiou, D. Kaplan, Porosity of 3D biomaterial scaffolds and osteogenesis. Biomaterials 26, 5474-5491 (2005).
2. B. Grigoryan et al., Multivascular networks and functional intravascular topologies within biocompatible hydrogels. Science 364, 458-464 (2019).
3. L. Tozzi et al., Multi-channel silk sponge mimicking bone marrow vascular niche for platelet production. Biomaterials 178, 122-133 (2018).
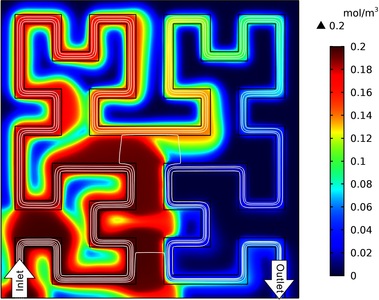
Herunterladen
- mu_poster.pdf - 1.56MB