3D Analyses of Changes in Free Bubble-Induced Stresses on Blood Vessel Wall in Ultrasound Therapy
Microbubble oscillations in the presence of ultrasound wave inside blood vessel have been widely studied for therapeutic applications like drug and gene delivery[1], blood-brain barrier opening[2], and lysis of blood clot[3] and cell membrane[4]. An oscillating bubble exerts stresses on the vessel wall. Circumferential[5] and shear[6] stresses on the vessel wall are believed to be responsible for vascular bio-effects. Recently, we have developed a photo-mediated ultrasound therapy (PUT) technology that uses combined ultrasound and laser irradiation to produce enhanced cavitation inside blood vessels. PUT has shown reduced perfusion rate[7, 8], which can treat medical conditions involving neovascularization. A numerical study has shown that PUT has three phases[9]. In the third phase, the bubble reaches a few micrometers in size starting from nanometer size in first stage and oscillates stably. In the third phase, there is no effect of laser and the only driving force is from the applied ultrasound. Moreover, the bubble may drift towards the vessel wall in the third stage.
Here, we have built a 3-D finite element-based model in COMSOL Multiphysics® to simulate the oscillations of free bubble in the third phase of PUT. A confined bubble inside a blood vessel oscillates differently than a free bubble. The confinement results in ellipsoidal shape and reduced oscillation amplitude. This model accounts for confinement effects of the vessel. In this model, an air bubble is assumed inside the vessel and surrounded by blood; the vessel is further surrounded by thick elastic tissue as shown in Fig (a). Blood is modelled as Newtonian & incompressible fluid and air is modelled as Newtonian & compressible fluid using the Laminar Two Phase Flow interface. The vessel and the tissue are modelled as linear elastic solid using the Solid Mechanics interface (Structural Mechanics Module). An ALE (Arbitrary Lagrangian Eulerian) based moving mesh is given to the fluid domain to account for the movement with time at vessel-blood interface and blood-bubble interface. A Fluid-Fluid physics interface with surface tension of 0.072 N/m is given at the blood-bubble interface and Laplace pressure (2*0.072/R) is added to the inner pressure of the bubble to balance the surface tension. A fully coupled Fluid-Structure Interaction coupling is applied at the blood-vessel interface. The outer part of tissue is given as perfectly matched layer (PML), to absorb the outgoing waves. The ultrasound wave is applied using boundary load physics at the vessel wall.
The model will be verified by comparing its results for large vessels with the Keller-Miksis equation results. The bubble dynamics and resulting circumferential and shear stresses on the vessel wall will be calculated using the model. Also, the changes in bubble dynamics and maximum stresses will be calculated for a bubble drifting towards the vessel wall by placing bubble in different off-center positions as shown in Fig (b). For a bubble drifting towards the vessel wall, the asymmetricity in bubble oscillation and maximum stresses on the vessel wall is expected to increase. The results from this study will be beneficial to understand the effect of an ultrasound activated off-center bubble in a blood vessel.
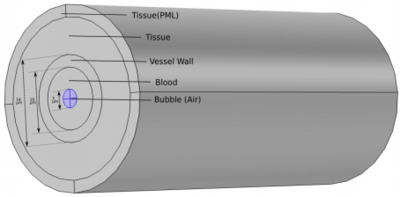